Many different types of batteries are used in the plant. The most common is the lead-acid battery. However, you will also find lead-calcium batteries and nickel-cadmium batteries. All storage batteries must be properly maintained in order to provide maximum service.
It is important to understand the characteristics of the industrial batteries used in the plant. Because the batteries are regularly discharged, they must be properly recharged to make them usable.
In this article we will explain the battery construction, discharging and recharging of the batteries, open cell voltage, specific gravity, and chemical action in battery cells.
Lead-Acid Batteries
The most common battery seen in the plant is the lead-acid battery. The negative and positive plates within the battery are made of lead and the electrolyte in the battery is sulfuric acid. Hence the name Lead-Acid Battery.
Led Acid Battery Cell Construction
Suppose you make a cell by placing two plates of pure lead in a jar and adding dilute sulfuric acid. Then suppose you connect a voltmeter between the plates. You will find that there is no potential difference between these electrodes. What you have made is not a cell. The reason is that the two electrodes are chemically the same. To produce electricity, the two electrodes must be made of different materials.
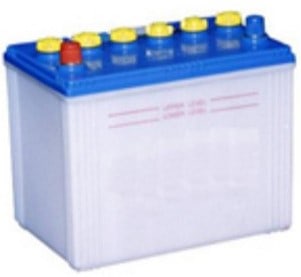
But suppose you go one step further. If you connect the plates to a source of direct current, electrons will flow into one plate, pass through the electrolyte, and leave the cell through the other plate. This current causes a chemical action involving both plates and the electrolyte. The chemical reaction changes the composition on the surface of the plates, making the two electrodes chemically different from each other.
Chemical Action In A Lead Acid Battery
Bubbles of hydrogen gas form at the plate attached to the negative terminal of the dc source. This lead plate becomes brighter in color than before. Bubbles of oxygen gas form at the other plate. This plate gradually changes from gray to dark brown. The brown material is a compound made of lead and oxygen. It is called lead dioxide (PbO₂).
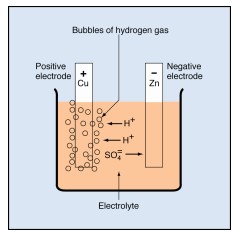
You have everything you need to form a cell once one of the plates changes to lead dioxide. You have two electrodes that are chemically different (lead and lead dioxide) in an electrolyte (sulfuric acid mixed with water).
If you test the cell only after a few minutes of connecting the dc source to the lead electrodes, you will find a potential difference of about 2V. The cell will produce enough current to make the lamp glow.
Chemical reactions between the electrodes and the electrolyte produce an excess of electrons on the lead electrode and a deficiency of electrons on the lead-dioxide electrode. This imbalance produces a potential difference between the electrodes, and it enables a current flow if the external circuit is complete.
If a test light is connected to a cell for long enough time, the light will gradually become weaker and eventually go out. Inside the cell, the dark brown lead-dioxide plate is now a lighter brown. The bright lead plate is a dull gray. At this point the cell is considered discharged.
The discharged cell cannot produce enough imbalance in electrons between the plates to drive current through the lamp circuit. A voltmeter shows that the potential difference between the electrodes is 0V.
The cell can be restored to back to operating condition by reconnecting it back to a DC source. It does take a while for the positive plate to become darker again and the negative plate to become shiny in appearance. The voltmeter test will now show the potential difference between the electrodes has been restored to about 2V. At this point, the cell is considered recharged.
Lead-Acid Battery Construction
Even though each simple lead-acid cell produces electric current, independently they do not have enough capacity to be a good source of electric power. The potential difference between the cathode and anode in each cell is only about 2V, and the current is limited by the size of the plates. The length of time that the cell will last before it needs recharged is limited by the amount of lead and sulfuric acid in the cell.
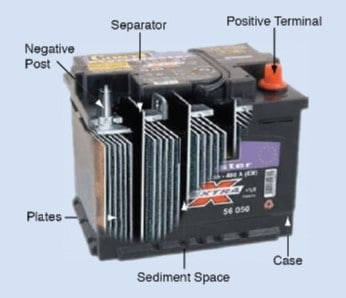
To produce the most amount of energy in the least amount of space, Several cells are connected together to produce a battery. Understanding the details of construction of a battery will help you understand how batteries work.
The positive and negative plates are made of an alloy of lead and antimony. An alloy containing 7 to 10% antimony provides greater strength and rigidity than pure lead. The alloy also provides greater resistance to corrosion by the acid.
The plates are often made in the form of a thin grid with open spaces between the lines of the grid. The grid is converted to a plate by filling it with a paste. The paste consists of about 25% lead and 75% lead oxide, mixed with water and sulfuric acid.
For the negative plates, the lead oxide is blended with about 1% of an expander to give it a larger volume. No expander is used for positive plates, but in all other ways the paste and manufacturing operations are the same as for negative plates.
After the plates have been pasted, dried, and assembled, they are immersed in weak sulfuric acid and charged electrically. In the negative plates, the chemical action during charging converts the paste to a porous plate of pure lead, called lead sponge. In the positive plate, the paste is converted to lead dioxide.
The amount of surface area exposed to the electrolyte has an important effect on the life of the battery.
• For some batteries, the plates are made thick enough so that grooves can be cut in the surfaces before charging. The grooves increase the surface area exposed to the electrolyte.
• For other batteries, only the negative plate is grooved. The surface area of the positive plate is increased by setting ribbons of lead in holes in a solid lead-antimony plate.
These two types of plates are used in stationary batteries, where is not as important as long life.
Battery plates are arranged parallel to one another, alternately positive and negative. All the positive plates are connected together by a metal strap. The negative plates are connected by another metal strap. The straps are connected to the battery posts, where connection is made to the external circuit.
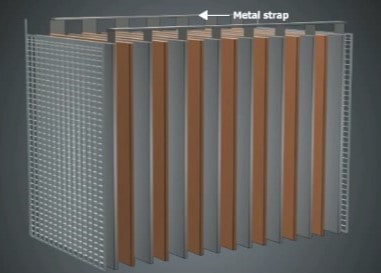
The length, width, thickness, and number of plates determine the capacity (in ampere-hours) of the battery. Batteries differ in these values to meet the requirements of different applications.
It is common practice to assemble the plates in a cell so that both outside plates are negative. Thus, the cell has one extra negative plate. A 15-plate cell has seven positive plates and eight negative plates. The two outside negative plates are thinner, because the outside surface of each one gets very little use.
Positive and negative plates must not come in contact with each other. Doing so would short-circuit the battery. The plates are kept apart by separators. Separators are usually sheets of wood, rubber, glass, or plastic. They are porous to permit the electrolyte to flow through them. The entire assembly of plates, straps, posts, and separators make up a unit called the element of the cell.
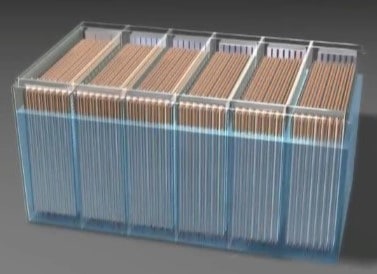
Several elements are placed in individual sections of a multicell container to make up a battery. The compartments of the container hold the electrolyte for each cell.
During operation, material flakes off the plates and falls to the bottom of each cell. This sediment gradually builds up until it causes a short circuit between the positive and negative plates. For this reason, the element is held up from the bottom of the container by a hard rubber or plastic grid called a bridge.
A cover is placed over the elements and sealed to the top of the container to keep out dirt and foreign matter and to reduce the evaporation of water from the electrolyte.
• On a soft-top battery, individual cell covers are installed and then the cell
connectors are welded between the terminal posts of adjoining cells. In this way the cells are connected in series. Finally the top of the battery case is sealed.
• A hard-top battery has a one-piece cell cover. This kind of cover reduces the formation of corrosive products on top of the case. Cell connectors pass through the cell partitions. Connectors and partitions are sealed so the electrolyte does not leak between cells.
Each cell has a vent cap so the electrolyte level can be checked and water can be added. The cap also allows gases to escape when the battery is charging.
Forming the Plates
When a dc source is connected to the lead plates in a lead-acid cell, a current flows through the electrolyte. The water in the electrolyte is broken down into its chemical elements— hydrogen and oxygen. This process of separating a compound into its elements by means of electricity is called electrolysis.
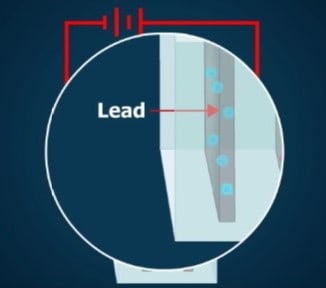
Every molecule of water is composed of 2 atoms of hydrogen and 1 atom of oxygen. Its chemical formula is H₂O. During electrolysis, the hydrogen atoms (H₂) recombine in pairs to make molecules of hydrogen gas (H₂). These molecules collect and form bubbles at the negative electrode. The bubbles escape from the electrolyte.
Some of the oxygen atoms (O₂) also recombine in pairs to make oxygen molecules (O₂) These molecules collect and form bubbles at the positive electrode. But most of the oxygen atoms combine with lead atoms (Pb) in the positive plate, forming molecules of lead dioxide (PbO₂).
Changing one lead plate to lead dioxide and leaving the other as pure lead is called forming the plates. After forming, the cell consists of two different electrodes—lead and lead dioxide. They are then ready to produce electricity in the cell.
Discharging
When the cell discharges, two other chemical reactions occur. In order to understand these reactions, you must first understand the nature of sulfuric acid. The chemical formula for sulfuric acid is H₂SO₄. However, the acid does not consist of molecules. Like all acids, sulfuric acid consists of ions.
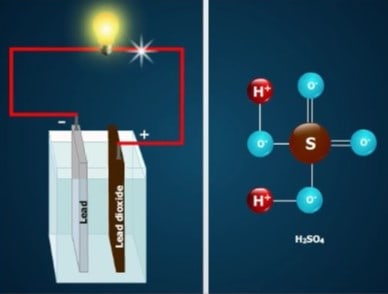
An ion is an atom, or a group of atoms, that has an electrical charge. That is, it has either too many or too few electrons compared to the number of protons.
There are two kinds of ions in sulfuric acid—positively charged hydrogen ions (H+), and negatively charged sulfate ions (SO=₄).
• The hydrogen ion is a hydrogen atom that lacks one electron. But a complete hydrogen atom has only one electron attached to one proton. Therefore, a hydrogen ion consists of just a single proton without any electrons.
• The sulfate ion consists of one sulfur atom combined with four oxygen atoms. The entire unit has two extra electrons, which come from the hydrogen ions, giving it a double negative charge.
During discharge, one chemical reaction occurs at the cathode (negative electrode) and another reaction occurs at the anode (positive electrode).
• At the cathode, pure lead combines with sulfate ions from the
electrolyte. Each combination is a molecule of lead sulfate (PbSO₄).
• The two extra electrons are released from the sulfate ion during this reaction. They enter the metal inside the cathode, creating an excess of electrons in the cathode.
• At the anode, the molecules of lead dioxide split apart. Each molecule produces two oxygen atoms and one lead atom.
• The two oxygen atoms combine with four hydrogen ions from the electrolyte, making two molecules of water.
• The lead atom combines with a sulfate ion from the electrolyte to form lead sulfate.
• The hydrogen ions accept electrons from the sulfate ions. But the sulfate ions cannot supply enough electrons for all the hydrogen ions. Additional electrons must come from the anode itself, creating a deficiency of electrons in the anode.
Recharging
Both reactions that occur during discharge can be reversed. To reverse them, you send a current through the cell in the opposite direction.
• The anode changes back to lead dioxide.
• The cathode changes back to lead.
• Water molecules in the electrolyte split apart, creating more hydrogen ions in the electrolyte.
• Sulfate ions leave both plates and return to the electrolyte.
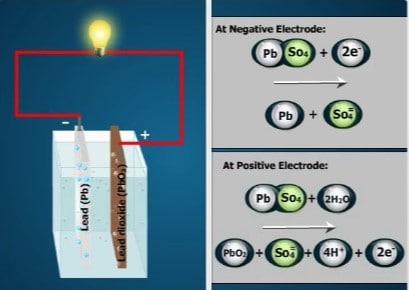
Lead-Calcium Batteries
One type of battery with a pasted-plate construction has grids made of pure lead plus about 0.07% calcium. Making the grids of nearly pure lead reduces internal losses in the battery. This reduction is important in batteries on standby service. These batteries require less charging and less attention, and they have a longer life. However, too much charging causes rapid deterioration of the plates, which shortens battery life.
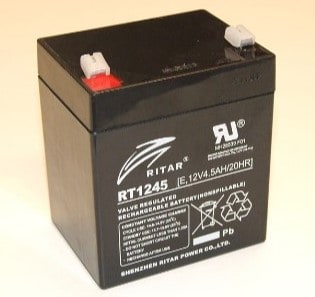
Cell Voltage
The potential difference between the plates in a cell depends only on the kinds of materials used in making the cell. Almost any two dissimilar metals in a conducting electrolyte produce a potential difference. But many combinations have no practical value. The lead-acid cell produces the highest potential difference—about 2V. A lead-acid battery with three cells produces 6V between the terminals. A six-cell battery produces 12V. Every additional cell adds 2V to the total.
The open-circuit voltage is the potential difference between the terminals when no load is connected to the battery, and is directly related to the of the electrolyte. The relationship can be written in the following way.
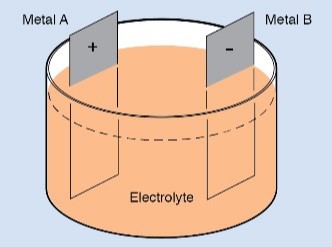
E = S.G. + 0.840
where E = potential difference, in volts
S.G. = specific gravity of the electrolyte
For example, suppose the specific gravity of the electrolyte is 1.210. The potential difference between the plates of this cell is 2.050 V (1.210 + 0.840 = 2.050). If the specific gravity is 1.280, the open-circuit voltage is 2.120V.
While a cell is delivering current, there is a drop in the potential difference between the terminals. This drop is caused by the cell’s internal resistance. The voltage drop increases with the discharge current.
At a continuous rate of discharge, the cell voltage gradually drops as the discharge progresses. As the cell nears depletion, the potential difference drops very rapidly to a value at which the cell is no longer effective. The value at the beginning of this rapid decrease is called the final voltage of the cell.
The final voltage varies with the rate of discharge. It may be as high as 1.85 V for low discharge rates, or as low as 1.0 V for extremely high discharge rates. A value of 1.75 V is used as the final voltage for most industrial batteries.
Specific Gravity
Many factors affect the specific gravity of the electrolyte when the battery is fully charged. First, the electrolyte must contain enough sulfuric acid. But if the specific gravity is too high, the electrolyte contains too much acid. A high concentration of acid can damage certain parts of the cell.
Other factors that affect the specific gravity of the electrolyte include the operating temperature and the age of the battery. The specific gravity at full charge may vary by 0.010 in either direction. The following examples indicate the full-charge specific gravities of batteries used for different purposes:
1.280 heavily worked or cycled batteries, as in electric industrial trucks
1.260 automotive batteries
1.220 stationary batteries in standby or emergency service.
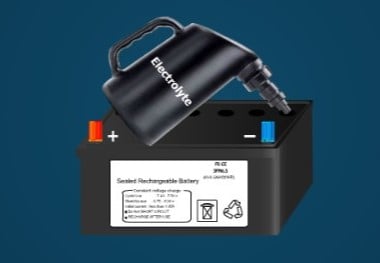
Capacity of Storage Batteries
The capacity of a storage battery is its ability to deliver electric charge. It is measured in ampere-hours. This rating is the discharge rate (measured in amperes) multiplied by how long the battery can continue to discharge (measured in hours).
This simple rating has very little meaning unless it is qualified by the many factors that affect a battery’s capacity rating.
• Discharge rate. The higher the discharge current, the lower the total number of ampere-hours delivered.
• Temperature. Higher temperature increases the rate of chemical reaction and increases the capacity of a battery. Lower temperature decreases battery capacity. The standard temperature for rating battery capacity is 25°C (77°F).
• Specific gravity. Higher specific gravity when the battery is fully
charged results in a greater capacity but a shorter battery life.
• Final voltage. The minimum useful voltage at which a battery delivers current varies with the discharge rate. It is lower at high discharge rates. The rated minimum potential difference for most lead-acid batteries is 1.75V.
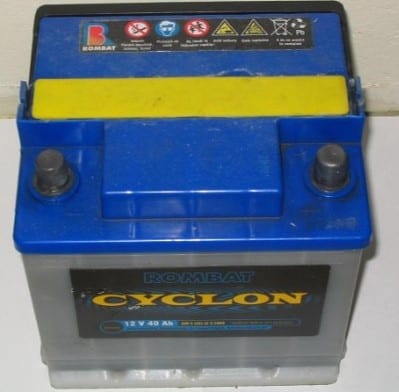
Nickel-Iron Alkaline Cell
The nickel-iron alkaline cell, also called the Edison cell, was invented by Thomas Edison. Nickel-iron alkaline cells once had many industrial uses. Today they have largely been replaced by the nickel-cadmium cell. However, you should be familiar with them because a few are still in use.
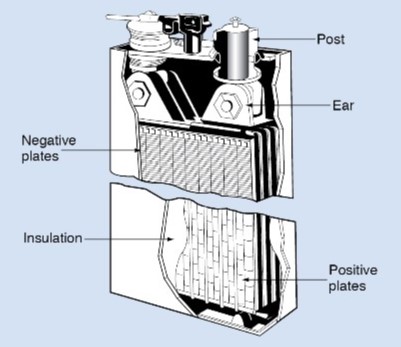
The nickel-iron cell had the same three basic parts as any other cell—a positive plate, a negative plate, and an electrolyte. In a charged cell, the positive plate consisted of nickel oxyhydrate [NiO(OH)(H2O)]. The negative plate was metallic iron powder (Fe). The electrolyte was potassium hydroxide (KOH) and water, with lithium hydroxide (LiOH) added.
The chemical reaction during discharge transferred oxygen from the positive plate to the negative plate.
• The positive plate changed to nickel hydroxide [Ni(OH)₂].
• The negative plate changed to iron hydroxide [Fe(OH)₂].
– The function of the electrolyte was to conduct hydroxyl ions (OH)₂ to the plates during discharge, and to accept the ions from the plates during recharging.
Construction of Nickel-Iron Cells
Positive plates were made of steel tubes packed with nickel hydrate and flakes of pure nickel. The tubes were perforated to allow the electrolyte to contact the nickel hydrate. Negative plates consisted of steel sheets shaped to form pockets. The pockets were perforated, and filled with fine iron powder.
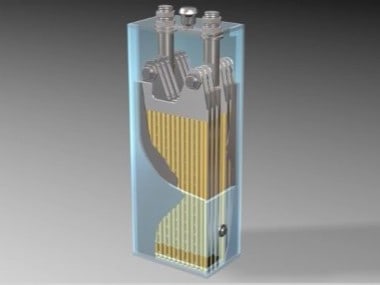
Groups of tubes or pockets were fastened together in a steel frame to form the positive and negative plates. The frames had a lug at the top with a hole in it to receive a connecting rod. The plate lugs were placed on the rod with spacing washers between them, and were held by nuts at both ends. The post for external connection to the circuit also fit on the rod. A complete assembly of either positive or negative plates was called a group.
Positive and negative groups fit together to form alternating positive and negative plates. Round insulators made of plastic or hard rubber fit between the plates. The assembly of positive and negative groups formed a unit called the element.
The container was made of nickel-plated sheet steel. It was an electrical conductor and had to be insulated from the element and from the posts where they passed through the cover. Insulation was provided by sheets of plastic or hard rubber. Hard rubber bushings called gland caps fit around the posts.
The entire assembly, plus the electrolyte, formed the cell. The cells in a battery were suspended on rubber buttons within the container. This arrangement provided ample ventilation to remove the heat generated during charging and discharging.
Voltage in Nickel-Iron Cells
Nickel-iron cells developed about 1.2 V. A ten-cell battery was considered to be a 12 V battery. The open-circuit potential difference between the terminals varied between 1.25 and 1.35 V. However, it was not closely related to the state of charge and could not be used to indicate when the cell needed recharging.
When the cell began discharging, the potential difference between its terminals immediately decreased, because of internal resistance in the cell. The voltage drop increased as the discharge current increased. At a constant rate of discharge, the potential difference gradually decreased until the cell was no longer effective. This final voltage was about 1.0 V per cell. It varied with the rate of discharge, and was lower at higher discharge rates.
Electrolyte in Nickel-Iron Cells
The specific gravity of the electrolyte of a new nickel-iron cell was about 1.210 to 1.215 at 25°C (77°F). The specific gravity slowly decreased over months or years from loss by splashing of the electrolyte during charging, and sometimes from accidental spillage.
When the specific gravity decreased to about 1.160, the electrolyte required renewal. This operation was necessary several times during the life of the battery.
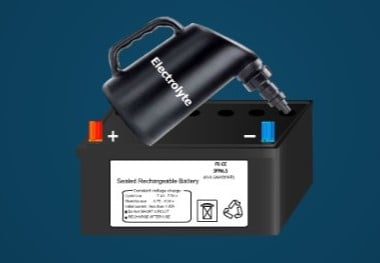
Potassium hydroxide (KOH) electrolyte for nickel-iron cells was furnished in two specific gravities.
• Electrolyte in the range of 1.200 to 1.210 was known as refill electrolyte. It was intended for replacing minor losses.
• Electrolyte with a specific gravity in the range of 1.240 to 1.250 was known as renewal electrolyte. It was intended for complete replacement.
The only time there was any significant change in the specific gravity of the electrolyte was when the discharge went beyond its normal limits near zero voltage, when cells were held in storage. During storage, much of the lithium passed from the plates into the electrolyte, raising the specific gravity 25 to 35 points (0.025 to 0.035). This action was reversed when the cells were recharged.
Nickel-Cadmium Cell
The nickel-cadmium cell is often called a nicad cell. It resembles the nickel- iron cell in many ways. The cadmium is substituted for iron in the negative plate, yielding several advantages.
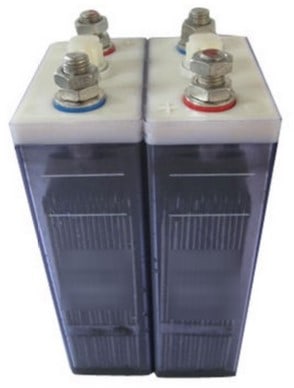
The most important advantage is a reduction in self-discharging. A nickel-iron battery lost its charge in three months if it was not recharged. A nicad battery retains 75% of its initial charge after a year of idleness. Another advantage is that the nicad battery can be fully charged by a very small . As a result, there is very little loss of electrolyte in a stationary battery.
Nicad storage batteries are built for two main kinds of service:
• High discharge rate applications. Examples include operating switchgear,
starting engines, providing emergency power, and other
applications requiring high current and minimum voltage drop
• Normal discharge rate applications. Examples include communications,
signaling, instrumentation, emergency lighting, and other uses.
Chemical Action in a Nicad Cell
The active ingredient in the positive plate is nickel hydrate [Ni(OH)H2O] with graphite to aid electrical conductivity. The active ingredient in the negative plate is metallic cadmium (Cd) with additives to aid conductivity.
The electrolyte is a solution of potassium hydroxide (KOH). Its specific gravity is 1.160 to 1.190 at 25°C (77°F). A small amount of lithium hydroxide is sometimes added to improve cell capacity.
The chemical reactions during charging and discharging are similar to those in the nickel- iron cell. The electrolyte takes no part in the chemical reaction, and its specific gravity does not change much during charging or discharging.
Construction of the Nicad Cell
Both positive and negative plates of the nickel-cadmium cell are the so-called pocket types. The basic construction of the two plates is identical. Pockets are formed from thin strips of perforated steel. Then the powdered active ingredients are poured inside. The nickel hydrate is mixed with powdered graphite instead of powdered nickel.
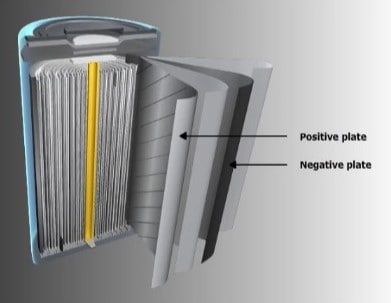
Individual pockets are connected together to form a plaque. The plaque is placed in a frame to form the plate. Plates of the same polarity are attached to terminal poles by a steel bolt and secured by a locknut. Steel washers assure proper spacing between the plates. This assembly is called a positive or negative group.
Positive and negative groups are then fitted together and insulators are inserted between the positive and negative plates. This complete unit is called the element.
The element is placed in a steel or plastic container. A cell cover with vent caps and holes for the terminals fits over the top of the cell. The space above the top of the plates serves as an extra reservoir for electrolyte.
Nicad Cell Characteristics
The potential difference between the terminals of a single nicad cell is 1.30 to 1.38 V. During discharge, the potential difference is about 1.2 V. Ten cells make up a 12 V battery.
When the cell is connected to a load, the potential difference falls to a value that depends on the rate of discharge and the state of charge. If the rate of discharge is constant, the potential difference gradually decreases until it reaches a point where the cell is discharged. This final voltage is about 1.14 V per cell. The value varies with the rate of discharge.
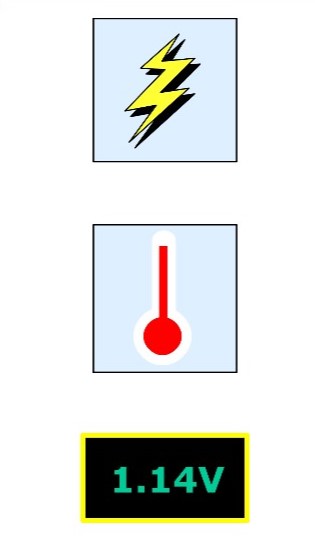
The capacity of the nicad cell is affected by three main factors.
• Discharge rate. The primary reason for the importance of this characteristic is the internal resistance of the cell. The higher the discharge rate, the greater the voltage drop and the lower the potential difference between the terminals of the battery. The final voltage is reached sooner at high discharge rates.
• Temperature. Chemical reactions take place faster at higher
temperatures. In addition the viscosity and resistance of the electrolyte are lower at higher temperatures. The effects combine to make the cell output greater at high temperatures than at low temperatures.
• Final voltage. At normal discharge rates, the final voltage is 1.14 V per cell. At very high discharge currents, the final voltage may be as low as 0.85V, and in some cases 0.65 V per cell.
Summary
The most common storage battery in the plant is the lead-acid battery. It is so named because its plates contain lead, and the electrolyte is sulfuric acid mixed with water. Other storage batteries used in the plant are the lead-calcium and the nickel-cadmium batteries.
All storage batteries require proper maintenance if they are to provide maximum service. Proper charging is essential when working with batteries. Lead-calcium batteries require less charging and less attention than lead-acid batteries, and they have a longer life. Their life, however, is greatly reduced by too much charging.
The capacity of a storage battery is its ability to deliver electric charge. A battery’s capacity is determined by its discharge rate, temperature, specific gravity, and final voltage.
The nickel-cadmium cell is often called a nicad cell. Its most important advantage is a reduction in self-discharging. It can also be recharged by a very small current.